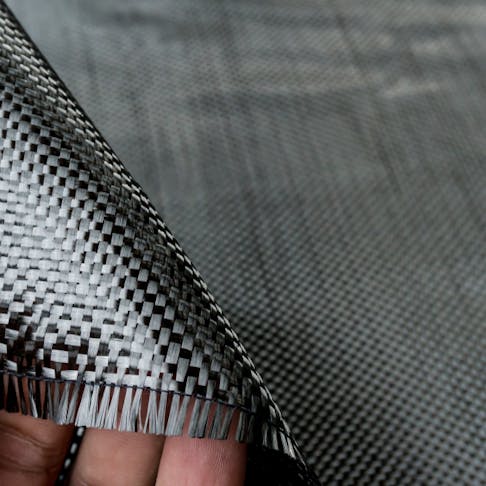
What is Composite Material? Definition, Properties, Types, and Applications
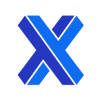
Composites are almost by definition characteristic of advanced technology applications—from race yachts to aircraft, skis to prostheses. They are symbolic of the leading edge of tech development. In reality, modern composites are new-material expressions of ancient techniques. The Mesopotamians were bonding strips of wood with primitive glues, alternating grain directions to make strong wooden structures in 3,400 BC, and the Egyptians were making cartonnage death masks by bonding papyrus strips with plaster or resin in 2,000 BC. This article will discuss what composite materials are, their properties, types, and applications.
What Is a Composite Material?
A composite material is made up of two or more materials with different chemical and physical properties. A composite material is used to enhance the properties of its base materials. Composites offer significant benefits in various material performance aspects, exceeding the mono-material alternatives and particularly standalone use of the constituent parts. Most man-made composites combine high tensile fibers that are flexible, with a matrix that forms the fibers into a rigid structure that acquires the compressive strength of the matrix material. The result is a combined material that benefits from the tensile strength of the fiber reinforcer, the compressive strength of the matrix, and the bending strength of their marriage to make a strong, rigid, stiff, and bend-tolerant resultant material.
What Is Composite Material Made Of?
Composite materials merge two (or more) divergent materials to produce a result that displays the advantageous properties of the constituents. Typically, the materials are referred to as the matrix, which is the bonding agent; and reinforcement, which is generally a fiber component. Typically the matrix materials are polymers, though they can also be ceramics and metals. The reinforcement is typically fibrous, formed from carbon, glass, or Kevlar® fibers/filaments/whiskers, though natural fibers are often integrated into composites. Figure 1 is an example of composite material:
Carbon fiber weave.
Image Credit: Composite_Carbonman
How Are Composite Materials Manufactured?
Manufacturing methods of composite materials vary widely. The key materials that are most commonly described as composites are glass, carbon, and Kevlar® fibers, bonded with resins. In the case of carbon fiber, the strands are pre-impregnated with a heat-activated polyester, vinyl ester, polyurethane, or epoxy resin (prepreg). The flexible sheets of woven fabric (roving) are laid up onto/into a mold and put under pressure. They are then heated to activate the resin which first liquifies, wetting all fibers, then curing to a tough, rigid result.
The pressure can be applied by tooling that closes and presses the fibers. Vacuum bagging can also apply pressure when a vacuum is applied to “pull” the shape into place. A third method employs an elastic pressure bladder that uses air pressure to “push” the shape into place. The end result, after the resin is cured, is a finished part with a shape that follows a mold faithfully, with little or no shrinkage, and a rigid, tough material that exploits the two components’ best properties. Similar methods are used with glass and Kevlar® reinforcers. The completed structure is then cured at room temperature or at a slightly elevated level and then released from the mold as a finished part or product.
Polymer composites and nanocomposites are very similar in their nature. They require other processes than the more widely recognized composites of carbon fiber and GRP (glass-reinforced plastic). Whether the end result material is a nanocomposite or a macro composite depends only on the scale of the additive—macro strands or nanomaterials. In both cases, the manufacturing method is essentially identical. The reinforcer material is considered an additive and is premixed with the polymer when the pellets are first manufactured. Molded parts have evenly distributed reinforcers throughout.
What Are the Properties of Composite Materials?
Properties of composites are as diverse as the range of materials that fall within this broad classification. Under ideal conditions, the resultant properties of the composite are:
- High strength/weight ratio.
- Impact resistant.
- Chemical/environmental stability.
What Are the Chemical Properties of Composite Materials?
Composites are often made with a rigid matrix of epoxy resin or a less rigid but still stiff matrix of a thermoplastic polymer. This component generally lends the below primary chemical properties to the resulting material:
- Can be designed for very high chemical stability. The selection of the matrix material and selecting non-absorbent and non-hygroscopic reinforcement fibers guarantee environmental resilience.
- Generally highly corrosion/environment resistant.
- Electrical and thermal conductivity can be engineered to any level from high breakdown voltage insulator up to moderate conductivity, by using appropriate additives and reinforcer materials.
- Achieving flame resistance or retardancy in composites is also a design property that can be highly controlled.
What Are the Physical Properties of Composite Materials?
Some common themes in the physical properties of composites can be generalized, including:
- The tensile strength of most composites approaches or exceeds that of the reinforcing material.
- The compressive strength is similar to or exceeds the compressive strength of the matrix material. This is despite the fact that the overwhelming bulk of most composites is a reinforcer and not a matrix.
- The bending strength generally greatly exceeds the tensile capacity of the matrix material, as the tensile loads of bending forces are transferred to the reinforcer component and dissipated without fracture.
- Electrical or thermal conductivity.
- Abrasion resistance.
- Reduced weight/density.
- Magnetic properties.
- Optical properties.
- Shock resilience.
- Fatigue and creep resistance.
How Does Composite Differ From Plastics?
Plastic components are generally made from a single polymer injection, or sometimes a two-stage process that overmolds rubber onto a part for specific applications such as grip and seal. They are relatively easy to manufacture, generally involving a single-stage operation. Composites, on the other hand, are always two or more co-processed materials, achieving better properties than the individual components can deliver. They are also intrinsically more complex to manufacture. They generally require manual lay-up processes and tend to be much more expensive in labor terms than simple, automatable molding operation outputs. Composites are generally intrinsically stronger than equivalent plastic parts by a large factor. This allows composite parts to deliver higher strength and reduced weight, compared with a similar plastic component.
Plastic parts are, in most regards, shape, and size unlimited. Composite parts are rarely used for very small components but can be very large, but they are quite restricted in the complexity of shapes and fine detail. Broadly, plastics are used for low-cost, high-volume applications, whereas composites are considerably more costly and are used for high-value and lower-volume tasks.
What Are Different Types of Composite Material?
The range of composites employed in manufacture/construction is extensive, but they fall into these broad categories. These are listed below:
1. Nanocomposites
Nanocomposites are both man-made and naturally occurring. The reinforcer is generally a nanomaterial such as carbon nanotubes or graphene added to a polymer matrix, or silicon nanoparticles added to steel to induce fine crystal growth. In some applications, calcium carbonate or talc can also be effective in making polymers stiffer and stronger.
Typical nanocomposites use the nanomaterial additive to add strength, stiffness, and other properties such as electrical or thermal conductivity to the polymer matrix. Naturally occurring nanocomposite examples are bone and shell. Nanomaterials represent significant health risks in some cases, so manufacture of these materials can be challenging.
2. Metal Matrix Composites (MMCs)
MMCs use a metal matrix like aluminum or magnesium and a high-strength fiber reinforcer in particle or whisker form. Reinforcers are generally carbon fiber or silicon carbide particles. This develops unique properties that go beyond the basic metal component’s limits, including: increased strength and stiffness, elevated temperature resistance before the onset of weakening, improved wear resistance, and reduced coefficient of thermal expansion.
MMCs are used in aerospace industries and extreme automotive uses, delivering high strength and low weight. They are also used in electronics, medical devices, and sporting goods. The processing of MMCs is more challenging than most other classes of composites, as high temperatures and the difficulties of uniform reinforcer distribution are challenging.
3. Polymer Matrix Composites (PMCs)
PMCs are the most prevalent and easily understood forms of composite materials. This term encompasses the hand lay-up of carbon fiber and glass fiber fabrics and the manual, injected, or pre-impregnated epoxies and polyester resins that form the matrix. These materials offer various benefits including: high stiffness and strength (compared with the part weight), great thermal, chemical, and mechanical resilience, and abrasion resistance. On the other hand, PMC requires highly skilled labor, resulting in higher costs, though these are often not excessive for applications that need a high-strength outcome.
PMCs are widely used in aerospace, automotive, marine, and sporting goods, benefitting from light weight, high strength, and stiffness. Production of PMCs involves assembly methods such as hand lay-up and filament winding, which can be slow. Precise control over the curing process is needed, to achieve ideal material properties.
4. Glass Fiber Reinforced Polymers (GFRPs)
GFRPs are a subset of polymer matrix composites, specific to epoxy and polyester bonded glass fiber materials. The glass fiber can be in chopped strands, lending a degree of anisotropic strength to structures by the mixed orientation of the fibers. The reinforcer can also comprise chopped strand roving (or fabric), making a more orderly process but less well suited to bulk components as fibers are all laid in one plane. Woven roving improves the quality of lay-up and can offer greater strength, at a price.
5. Hybrid Composites
Hybrid composites are those in which two or more different reinforcing fibers are integrated into the final material. This could be a combination of glass and carbon fiber in a lay-up—for enhanced impact resistance or cosmetic reasons. It is common to use titanium mesh or strands in the manufacture of racquets for ball sports, to improve tensile and bending performance. These materials can be challenging, as compatibility issues can affect the behavior of the material—for example, one fiber may bond better to the matrix than the other. Considerable testing is required to confirm the value or feasibility of the hybrid matrix. They have the same applications as PMCs, but the higher cost restricts their use.
6. Ceramic Matrix Composites (CMCs)
CMCs consist of a ceramic matrix and reinforcing fibers. A ceramic matrix provides extreme temperature and corrosion resistance and excellent wear properties. But ceramics are generally brittle when unreinforced. The addition of silicon carbide, alumina, or carbon fibers can counter the brittleness to make a more serviceable material.
CMCs are used to make gas turbine blades, specialist rocket/aerospace components, and heat exchangers. CMCs are very costly and they remain quite brittle, which limits their use. However, this is a field of intense research, and properties are improving.
7. Natural Fiber Composites (NFCs)
There is an increasing trend toward using natural fibers in composite manufacture, to reduce the environmental impact of materials use. Natural fibers such as jute, flax, cotton, and wood are used in a variety of ways. Automotive interior panels are commonly made from resin-bonded natural fibers which are compression molded to shape and then upholstered in plastics or leather for final surfacing. Wood fibers are added to polymers for FDM/FFF rapid prototyping filaments, to improve strength and produce a wood effect. Skateboard decks make extensive use of natural fiber reinforcement, generally in a polyester resin matrix.
8. Carbon Fiber Reinforced Polymers (CFRPs)
CFRPs are another subset of polymer matrix composites, specific to epoxy and polyester-bonded carbon fibers. For hand lay-up purposes, carbon fiber is generally used as woven roving, with a range of weave patterns used for various types of loading and stress distribution. The fibers are pre-impregnated with thermally activated resins, so the flexible cloth is laid-up and then compressed and baked to liquify and then cure the resin to create a rigid, tough result. Carbon fiber can also be pultruded with a range of polymers, to make continuous lengths of CFRP in complex sections.
9. Aramid Fiber Reinforced Polymers (AFRPs)
AFRPs are another subset of polymer matrix composites that employ aramid as the reinforcer. Aramid fiber composites are used in the highest-impact applications. The aramid is generally used as woven fabrics that are pre-impregnated with appropriate epoxy and polyester resins, to be processed as per carbon/glass fiber. Another aramid reinforced composite is the paper/aramid honeycomb material used in low-profile flooring panels in aviation—layered with aluminum sheets and epoxy bonded, this is a typical high-value hybrid composite.
10. Functionally Graded Composites (FGCs)
FGCs are essentially a subset of any type of composite. These are composite materials in which the constituent parts can be modified in the application or type through the structure to tune performance. A gradual transition in properties is used to avoid stress concentrations at sudden changes. The functional grading can be: as simple as adding or altering fiber content at elevated stress points; changes in weave pattern in roving to alter load distribution; or progressive hybridization for impact resilience in regions.
FGCs are used to make lighter and more resilient aircraft and spacecraft components, such as turbine blades and rocket nozzles. Biomedical devices/implants can have varied properties regionalized according to desired tissue interactions.
What Type of Composite Material Is Used for 3D Printing?
A range of additive materials is integrated into various polymer 3D printing materials for FDM/FFF, SLS, and other systems. The resultant materials offer enhancement of properties such as tensile strength, bending strength, hardness/abrasion resistance, etc. Examples of 3D printable composites are
- Chopped carbon
- Glass
- Kevlar®
- Wood fibers
- Carbon fiber
What Are the Advantages of Using Composite Materials in 3D Printing?
Fiber and metal additives in 3D printing materials offer some potential advantages. These are listed below:
- Deliver increased strength and stiffness, allowing more functional printed outcomes or reduced weight for the same strength.
- Can be more durable than matrix material alone. This may enable parts to function at higher temperatures.
- Some composite additives can add electrical or thermal conductivity or increase breakdown voltage.
Some 3D printing processes use a form of functional grading, by co-printing rigid and elastomeric materials in the same parts, allowing properties to be varied through a build.
What Are the Disadvantages of Using Composite Materials in 3D Printing?
There are challenges in using composites in some 3D printing processes, as listed below:
- Additive-loaded materials can bring processing challenges. For example, they can be difficult to shape using established technologies.
- Few options are on the market as yet, so availability can be a challenge.
- Highly functional composite print materials are more costly than their basic alternatives.
What Type of Composite Material Is the Most Ductile?
There is ongoing research into ductile magnesium matrix materials with titanium reinforcement. There is also ongoing development of metal-organic framework (MOF) polymer hybrids based on poly(urethane urea) which are reported as highly ductile. Ductility is not a common property in composites, as they tend to be a merger of a rigid, often brittle matrix and a flexible and elastic reinforcer. A prime example of this is ferroconcrete, which has virtually no ductility.
What Type of Composite Material Is the Most Brittle?
Ceramics are intrinsically brittle, and ceramic composites only marginally reduce this tendency. Ceramic turbine blades have great thermal performance but rarely exhibit good impact resilience. For more information, see our guide on Brittleness.
What Are Examples of Composite Materials?
There are hundreds of composite materials in daily use. These are some examples:
- Glass-reinforced polyester resin.
- Epoxy-bonded carbon fiber.
- Plywood in all its forms.
- Ferroconcrete.
- Glass-reinforced ABS, PEEK, nylon.
- AeroBASE.
- Plastic molded over a metal structure for comfort/aesthetics.
What Are the Different Applications of Composite Materials?
Listed below are some applications of composite materials:
- Motorcycle fairings, kayaks, boat hulls, and aircraft skins.
- Epoxy-bonded carbon fiber in fishing rods.
- Plywood for construction.
- Ferroconcrete for construction.
- Glass-reinforced plastic for high-strength molding.
- Aircraft flooring, sandwiching paper honeycomb between two aluminum sheets.
- Spectacle frames (often mold plastic over a metal structure).
How Are Composite Materials Used in 3D Printing in the Aerospace Industry?
The aerospace sector is always looking for higher strength and lower weight. The ability to directly manufacture parts with carbon fiber or even have continuous-strand fibers built-in offers opportunities to achieve remarkable strength levels in low-volume parts of unlimited complexity.
This is an experimental field that is not yet widely developed for making airworthy or certified manufactured parts—but this goal gets closer all the time.
Do Composite Materials Actually Work in 3D Printing in the Aerospace Industry?
No passenger-carrying aircraft will get an airworthiness certificate if built from or using 3D-printed parts in any flight-related function. This is irrespective of the materials being composite. In drones/UAVs however, extensive use is made of such parts in development and flight testing. As a rule, serviceability issues will rule such parts out from mass production.
Summary
This article presented composite materials and explained what they are, and discusssed its types, properties, and applications. To learn more about composite materials, contact a Xometry representative.
Xometry provides a wide range of manufacturing capabilities, including 3D printing and other value-added services for all of your prototyping and production needs. Visit our website to learn more or to request a free, no-obligation quote.
Copyright and Trademark Notices
- Kevlar® is a trademark of E. I. DuPont de Nemours and Company
Disclaimer
The content appearing on this webpage is for informational purposes only. Xometry makes no representation or warranty of any kind, be it expressed or implied, as to the accuracy, completeness, or validity of the information. Any performance parameters, geometric tolerances, specific design features, quality and types of materials, or processes should not be inferred to represent what will be delivered by third-party suppliers or manufacturers through Xometry’s network. Buyers seeking quotes for parts are responsible for defining the specific requirements for those parts. Please refer to our terms and conditions for more information.
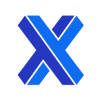